PowerPhotonic
PowerPhotonic has developed a process for manufacturing custom freeform optics at a competitive price. The ability to source optical elements, which are not limited by symmetric constraints, opens up the design space. I have worked with them to explore some of the application areas which are opened up by the possibilities that their process offers. The work described below was presented in a paper at Photonics West 2014. [1]
Beam shaping
In laser machining systems, the efficiency of the machining process can often be improved by transforming the beam from its initial shape at the machining interface. The configuration of a typical optical system for beam shaping is shown here.
There are a number of different approaches of using beamshapers and the advantages and disadvantages of the various techniques are discussed in the literature [2]. A number of different types of beam shaper can be generated using the PowerPhotonics process including:
Field mappers
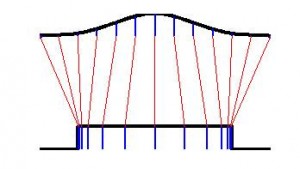
Field mappers are useful for well defined input and output beams and move energy from one part of a beam to another as illustrated schematically in Figure 2. They are particularly useful for coherent input beams such as from high energy low M2 laser beams where they are often used to transform beams from a Gaussian to a flat top beam. This transformation into either a circular or square top hat beam has been described in detail by Dickey et al [2].
Design of the field mapper was carried out in Matlab using the exact equations derived by Dickey. To confirm that it performed as expected, the design was then imported into Zemax and placed in a typical optical system.The figure below shows the expected beam profile before and after the field mapper and shows the transformation of a Gaussian input beam into a circular flat top output beam.
Figure 3: Zemax simulations showing the transformation of a Gaussian to a flat top beam
Microlens arrays
For incoherent beams, a fly’s eye array can be used to homogenise the beam as shown in Figure 4. In this approach to beam homogenisation, the input beam is carved up and each section is superimposed in the image plane.
This beamshaper comprises two lens arrays used in tandem, with the second array positioned in the focal plane of the first array. The purpose of the first array is to direct all the light from a single cell onto the corresponding lens in the second array. The second lens images the first microlens to infinity and therefore the far field image is a top hat with the shape of the lenslets in the first lens array.
Using the Powerphotonic process, square, rectangular and hexagonal lens arrays can be generated. In addition, as the transition zone between individual lenslets is a smooth surface, the losses from scattering are reduced compared to lens arrays made from standard processes. An example of a hexagonal lens array designed and made is shown in Figure 5 below:
Figure 5: Hexagonal lens array generated by the Powerphotonic process
Pseudorandom Refractive Intensity Mapping Elements (PRIME)
One advantage of the Power Photonic process for the designer is that the process generates a smooth profile over the surface. Each point on the surface is generated independently of each other and so a completely freeform surface is possible. This has opened up the option of designing a pseudorandom refractive surface. A technique has been described by Dixit et al [3].
This is an iterative Fourier transform(IFT) technique, similar to that used to design diffractive optical elements, but without discretization of phase. In the technique, phase discontinuities and hence phase branching are forbidden. In Diffractive optical elements (DOEs), these allow greater design flexibility, but contribute to scatter loss. The refractive designs begin with a pseudorandom surface, which is iterated until it produces the required far-field profile. Although the design process is based on a diffraction calculation, the resultant optic is a refractive device, mapping intensity from small cells in the input beam over the whole of the output beam. The design is carried out using Matlab to generate the surface and the optic is then imported into Zemax and checked there.
PRIME optics directly tackle the main deficiencies of DOEs. Their most relevant properties in this regard are:
- High efficiency: no diffraction losses
- Very low scatter: due to the ultrasmooth refractive surface
- No zeroth order leakage: These beamshapers can be used on axis, and integrated into standard process head architectures
- High laser damage threshold: PowerPhotonic’s fabrication process results in ultrasmooth surfaces that are particularly resistant to laser damage, and the smooth, slowly-varying surface avoids generation of local hotspots in the beam
- Wavelength independence: These are refractive optics, and the gross behavior is wavelength-independent. The exact structure of speckle is wavelength dependent when used with sources with high spatial coherence.
Figure 6: Example of a PRIME optic designed and then manufactured by Power Photonics
The images below show the designs as generated in Matlab and then imported into Zemax.
These beamshapers were designed to transform a Gaussian input beam into a flat top beam in the far field. An experiment was carried out by PowerPhotonics to test the performance of the beam shaper. The source was a fiber-coupled red diode laser, wavelength 635nm, delivered via a 1m fibre with a core diameter 62.5µm and NA 0.275, resulting in a beam diameter of approximately 11mm after the collimating lens. The modal properties of this source are particularly ugly, as shown in Figure 8. The output beam exhibited strong speckle, which was very sensitive to movement of the delivery fiber. This is a typical result of launching a spatially coherent source into an overmoded fibre.
The output beam generated by the beamshaper is shown in Figure 9. The first column shows the output beam with a static delivery fibre. The design beam profiles are produced as intended, with a fine speckle structure superimposed, due to the high spatial coherence of the source. A source of lower coherence (uniform mutually incoherent filling of fiber modes) was simulated by vibrating the fiber. The result is shown in the second column of Figure 9. This shows a much smoother output intensity profile. Despite the poor correspondence between the Gaussian beam used in the design and the actual input beam, the beamshaper succeeded in generating a good quality flat top output beam.
References
- Roy McBride, Natalia Trela, Matthew O. Currie, Duncan Walker, Howard J. Baker Beamshaping for high-power lasers using freeform refractive optics, Photonics West LASE 8963-12 (2014)
- Fred M. Dickey, Scott C. Holswade, “Laser Beam Shaping: Theory and Techniques”, CRC Press, (2002)
- Designing fully continuous phase screens for tailoring focal-plane irradiance profiles S. N. Dixit, M. D. Feit, M. D. Perry, and H. T. Powell. Vol. 21, No. 21 Optics Letters Vol 21, No. 21 1715